Early microscopists relied on oil lamps and natural sunlight to provide an external source of illumination for their primitive (but often remarkably accurate) microscopes. They often employed rather ingenious methods of gathering light, such as reflection from a large white board or scattering of sunlight on a cloudy day. Unfortunately, these methods did not provide reliable illumination and frequently the area of field illumination greatly exceeded the numerical aperture of the objective, causing glare and flooding.
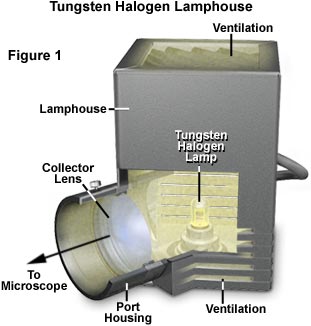
Modern microscopes usually have an integral light source that can be controlled to a relatively high degree. The most common source for today's microscopes is an incandescent tungsten-halogen bulb positioned in a reflective housing that projects light through the collector lens and into the substage condenser. Lamp voltage is controlled through a variable rheostat that is commonly integrated into the microscope stand. A typical illuminator lamp and housing is illustrated in Figure 1. The bulb is a tungsten-halogen lamp that operates on a direct current (DC) voltage of 12 volts and produces up to 100 watts of power for illumination. Lamp voltage is controlled by a DC power supply that is often built into the microscope housing, with a voltage control knob that is usually a potentiometer mounted somewhere on the microscope stand. These bulbs generate a large amount of heat during operation, and the housing is provided with several layers of heat sinks to help dissipate excess heat. The position of the lamp is controlled by a series of knobs on the side of the illuminator housing or is pre-centered specifically for the housing. Light from the lamphouse is directed into the microscope base through a collector lens (Figure 1), and then frequently through a sintered glass diffuser before being focused on the aperture diaphragm of the condenser.
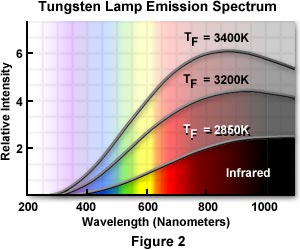
Incandescent Lamps - Incandescent tungsten-based lamps are the primary illumination source used in modern microscopes, with the exception of those intended for fluorescence microscopy investigations. These lamps are thermal radiators that emit a continuous spectrum of light extending from about 300 nanometers to upward of 1200-1400 nanometers, with a majority of the wavelength intensity centered in the 600-1200 nanometer region as illustrated in Figure 2. Their design, construction, and operation is simple consisting of an enclosed glass bulb filled with an inert gas and containing a tungsten wire filament that is energized by a DC electric current. The bulbs produce a tremendous amount of heat and light, but the light accounts for only 5 to 10 percent of their energy output. Tungsten lamps (but not tungsten-halogen) are similar in operation to common household light bulbs and likewise tend to suffer several drawbacks such a decreased intensity with age and a blacking of the inside envelope as evaporated tungsten is slowly deposited. The color temperature and luminance of these lamps varies with the applied voltage, but average values range from about 2200 K to 3400 K. When these lamps are used in photomicrography with color film, the microscopists must use a lamp voltage that produces a color temperature matching that of the film emulsion, usually somewhere in the range between 3150 K and 3250 K. Often, the color temperature must be fine-tuned for photomicrography by inserting filters into the light path that balance the illumination for the color temperature of the film emulsion.
Lamp Filament Alignment
Explore how the lamp filament is aligned to achieve Köhler illumination.
Tungsten lamps vary widely in their design and the diverse set of models offered by manufacturers feature a variety of envelope shapes, mounting fixtures, and filament arrangements. A typical selection of tungsten lamps used in optical microscopy is illustrated in Figure 3. The bulb in Figure 3(a) is a 6-12 volt square tungsten filament with a bronze bayonet base that is designed to be used with the end of the cylindrical bulb facing the collector lens. The rounded-envelope bulb illustrated in Figure 3(b) also has a bronze bayonet base, but this less-powerful 6 volt bulb can be positioned to project light either sideways or end-on. The bulb in Figure 3(c) also has a rounded envelope, but is equipped with an Edison screw-thread base. With an operating voltage between 6 and 30 volts, this bulb is designed to be used end-on.
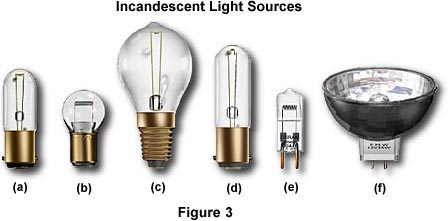
The bulb illustrated in Figure 3(d) is very similar to that in Figure 3(a), with the exception of having an extended glass envelope. It is equipped with a brass bayonet base and is a common design used in a number of microscopes built in Eastern Europe. A modern tungsten-halogen lamp (sometimes referred to as a quartz-iodine bulb) is illustrated in Figure 3(e). These lamps are now standard equipment on most microscopes manufactured in Japan, the United States, and Western Europe. Tungsten-halogen lamps have compact bulbs that introduce a number of advantages over normal incandescent lamps, most notably their brilliant light, smaller dimension, uniformity of illumination, longer lamp life and greater economy. Unlike tungsten-filament incandescent lamps, tungsten-halogen lamps have halogens added to the filler glass. The halogens (usually iodine) ensure that all vaporized tungsten is returned to the filament and not deposited upon the glass envelope.
CAUTION! |
---|
Tungsten-halogen lamps operate at very high temperatures and may cause serious burn injuries if handled while hot. When replacing these lamps, always allow them to cool for at least 20 minutes before removing them from the lamphouse. Avoid handing the bulb envelope directly because fingerprints left on the envelope will become burned into the glass, often initiating premature lamp failure. Manufacturers package tungsten-halogen lamps in protective plastic bags to avoid handling problems. Use a pair of scissors to cut the bag near the tungsten pins and insert the lamp into its holder while it still remains in the bag. Remove the bag when the lamp is properly positioned in the lamphouse. |
The filaments of tungsten-halogen lamps are often very compact arrays mounted in a borosilicate-halide glass (often termed "fused quartz") envelope. Operating voltages for these lamps range from 4-24 volts with power ratings from 20-100 watts. They have very high filament operating temperatures, restricting their use to well-ventilated lamphouses with fan-shaped heat sinks to eliminate the tremendous amount of heat generated by these bulbs. The base is a two-pin style with tungsten lead wires fused to the borosilicate glass envelope. Illumination from tungsten-halogen bulbs is remarkably uniform throughout the bulb life, which can range from 1000-2500 hours. Tungsten-halogen bulbs emit a continuous spectrum of light with a color temperature ranging from 2700-3350 K (depending upon voltage), although there is some decline in the color temperature value as the bulbs begin to age.
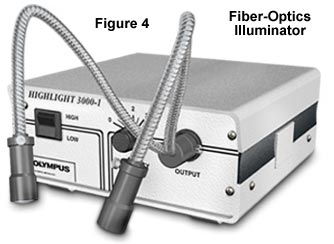
The lamp illustrated in Figure 3(f) is a tungsten-halogen dichroic reflector that is commonly used in fiber-optics light sources, like the one pictured above in Figure 4. The reflector aids in directing the light by allowing most of the infrared radiation generated by these bulbs to pass through the dichroic mirror reflector housing, while reflecting the shorter visible and near-ultraviolet wavelengths. These lamps operate at 6 to 21 volts DC and are usually placed in a lamphouse that provides for control of the operating voltage as well as removal of excess heat through cooling fans. Illuminators using these lamps are often termed "quartz-halogen" fiber optic lamphouses that provide high intensity illumination, but have the downside of producing a significant amount of infrared light in the form of heat. Direct lighting on a specimen will generally result in a high degree of heat absorption by the specimen, and care should be taken to ensure that this does not lead to degradation. The lamphouse illustrated in Figure 4 contains lens elements on the ends of the fiber optic tubes that allow the light to be focused onto the specimen.
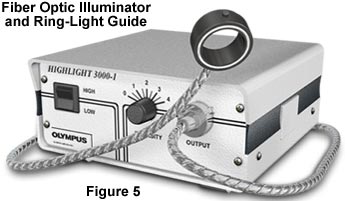
Also available are other peripheral components such as colored glass filters to produce special effects lighting and polarizers that attach to the lens elements to allow illumination with polarized light. These light sources are often used as auxiliary oblique illumination for reflected light microscopy with compound and stereo microscopes. Specially designed ring illuminators, as depicted in Figure 5, can be used to create a bright, 360° cool white, shadow-free even-intensity illumination. They are designed to mount directly to stereo microscope objectives and are useful even with high-magnification and long working distance (1.5 to 10 inches) objectives without light adjustment when refocusing or when zoom features are used. Polarizing units are manufactured for the ring-light guides so that light reflected back into the objective can be polarized. There are also glass and gelatin filter kits that can be used with these lighting attachments.
Microscopes not equipped with an internal source of illumination must resort to external light sources to provide illumination for the sample. A typical external illumination source is illustrated in Figure 6. These illuminators may contain a variety of incandescent tungsten lamps including both 6-12 volt DC lamps as well as 120 volt coil-filament lamps that sport power ratings from 25-100 watts.
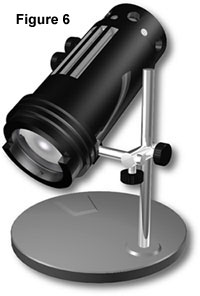
Direct current-powered versions of the external illumination source depicted in Figure 6 often have an external power supply that converts 120 volt wall power into the necessary 6-12 volts DC required by the lamp. These sources also are usually equipped with diffusers to even out the light intensity as well as filters to help with color balance. Some models also provide space for a polarizer so that polarized light microscopy can be conducted with the external lamp. The lamps often have a "field" diaphragm that allows the microscopist to adjust the diameter of the light beam entering the microscope. External light sources focus light onto a substage mirror that must be carefully adjusted to reflect light into the condenser at the proper angle to allow light entering the objective to be "centered" within the optical axis of the microscope.
The Field Diaphragm
Discover how the field diaphragm controls the amount of light entering the microscope.
Arc Lamps - Mercury vapor, xenon and zirconium arc lamps are also useful sources of illumination for specialized forms of microscopy. These lamps are controlled by external power supplies that are designed to meet the electrical requirements of first igniting the lamp, then providing the correct current to maintain constant illumination. Several typical arc lamp designs are illustrated in Figure 7. The lamp in Figure 7(a) is a mercury vapor lamp equipped with an igniter electrode and the lamp in Figure 7(b) is a modern HBO 200 watt mercury short arc lamp powered with alternating current through an external power supply. This and other similar arc lamp power supplies will furnish enough start-up power to ignite the burner (by ionization of the gaseous vapor) and keep it burning with a minimum of flicker.
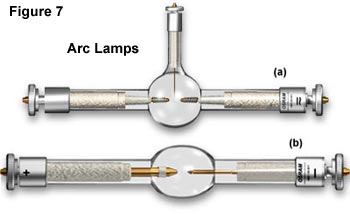
Arc lamps have an average lifetime of about 200 hours and most external power supplies are equipped with a timer that allows the microscopist to monitor how much time has elapsed. Mercury arc lamps (often referred to as "burners") range in wattage from 50 watts to 200 watts and consist usually of two electrodes sealed under high pressure in a quartz glass envelope which also contains mercury. These arc lamps do not provide even intensity across the spectrum from near-ultraviolet to infrared (see Figure 8 for the emission spectrum of the mercury arc lamp).

Much of the intensity of the mercury arc lamp is expended in the near-ultraviolet, with peaks of intensity at 313, 334, 365, 406, 435, 546, and 578 nanometers. These lamps are not generally useful for most forms of microscopy (with the exception of fluorescence microscopy), but serve as excellent monochromatic light sources for black and white photomicrography. Using the appropriate filters, the green line at 546 nanometers, the blue line at 435 nanometers, and the near-ultraviolet line at 365 or 406 nanometers can yield excellent monochromatic light in selected wavelength regions. A mercury vapor arc lamp should never be used for brightfield, darkfield, DIC, or polarized color photomicrography because the limited emission spectrum of the lamps will not yield true color renditions of the specimen.
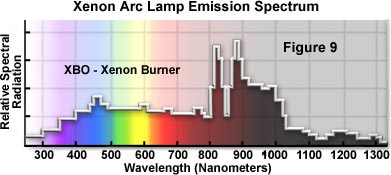
The xenon arc lamps have much more even intensity across the visible spectrum than do the mercury vapor lamps (see Figure 9); they do not have the very high spectral intensity peaks that are characteristic of the mercury lamps. Xenon lamps are deficient in the ultraviolet; they expend a large proportion of their intensity in the infrared, and therefore the use of such lamps requires care in control of heat. Short-gap xenon burners are usually more desirable because the size of the arc is such that its light may be much more readily included within the back aperture of the objective, thus avoiding waste of light intensity.
CAUTION! |
---|
Mercury and Xenon arc lamps require caution during operation because of the danger of explosion due to very high internal gas pressures and extreme heat generated during use. Never ignite a lamp outside of its housing or observe the lamp directly when it is burning (this can cause serious eye damage). Neither mercury nor xenon lamps should be handled with bare fingers in order to avoid inadvertent etching of the quartz envelope. Change bulbs only after the lamp has had sufficient time to cool. Store lamps in their shipping containers to avoid accidents. |
Always adhere to the safety procedures listed above when installing or changing mercury or xenon arc lamps. Mercury arc lamps, as described above, have a life of about 200 hours; xenon burners several hundreds of hours. Frequent on-off switching reduces lamp life. When the burners reach their rated lifetime, the spectral emissions may change and the quartz envelope weakens.
Zirconium arc lamps are another excellent source for microscope illumination. They provide a very small (almost a point source) beam of light that has a color temperature of about 3200 K. While these lamps do not emit light as bright as the mercury or xenon arc lamps, they do provide a continuous spectrum of light that is suitable for photomicrography using color film.
Laser Light Sources - In recent years, there has been increasing use of lasers, particular the argon-ion laser with powerful emission capability at 488 and 514 nanometers. Laser sources, despite the high cost, have become especially useful in laser scanning confocal microscopy.
Laser Light Sources
Explore how a ruby laser is excited by a xenon flash tube to produce coherent red light.
There are a number of different types of lasers that each provide a unique emission spectrum. Figure 10 illustrates the emission spectra of the two most commonly used lasers in microscopy (fluorescence, confocal, and monochromatic brightfield).
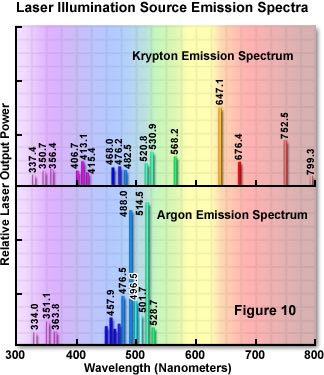
Electronic Flash - A specialized method for photographing moving specimens (especially in darkfield illumination) has been devised using electronic photography flash systems. These flash tubes provide 5500 K illumination in an instantaneous burst that can capture great specimen detail when used with high speed (ISO 400 and above) daylight transparency film. These units must be accompanied by a continuous source of tungsten illumination to ensure framing of the specimen, Köhler illumination, focusing, and alignment of the microscope prior to the flash photomicrography. Flash tubes should be synchronized to the camera shutter of the microscope, and a number of manufacturers provide this equipment as an accessory for photomicrography.